Modern Point-of-Care diagnostic devices rely on microfluidic technology to analyze the smallest amount of fluid, for the highest measurement precision possible. To do so, the microfluidic devices require powerful tools to detect and measure targeted elements (e.g., pathogens). This can be achieved by coating the microfluidic channel walls with the appropriate molecules (e.g., antibodies) to capture the target (Figure 1). These biological sensors are then coupled with electrical sensors, embedded inside the microfluidic device, to translate a capture event into readable data.
In this article, we discuss the very first step of microfluidic channel coating, which consists of modifying the surface chemistry of the microfluidic channel wall, before depositing the actual sensors. Here, we recapitulate the common surface functionalization methods applicable to microfluidic technology. We especially focus our attention on devices prepared from elastomeric polymers (e.g., SEBS) like FlexdymTM, which are cheap and easy-to-handle materials perfect for microfluidics in academia, as well as in industry. Read to find out how you can perform the surface treatment you need to develop your own sensors, without the need for an advanced chemical engineering degree!
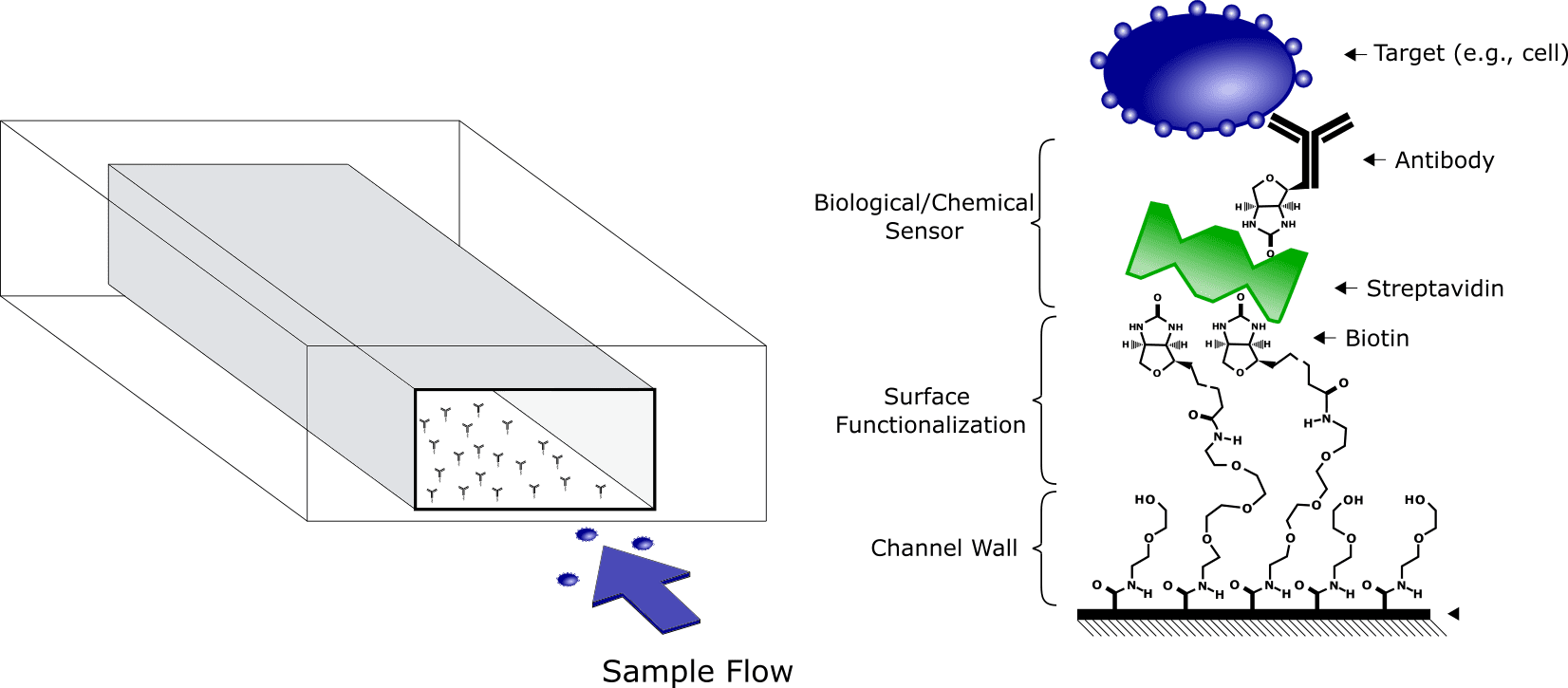
Figure 1 : Example of a Surface functionalization on microfluidic channel wall : The surface functionalization is terminated with a biotin molecules, which is then bonded to a streptavidin molecule coupled with an antibody as biological sensor/trap.
Microfluidics surface functionalization : A must-have for Point-of-Care Diagnostics
Nowadays, using microfluidic systems with functional patterned surfaces is an interesting strategy to construct polymeric platforms with detection capabilities. Microfluidic devices have a great potential to be widely used as diagnostic methods for many diseases, especially in remote areas where well-equipped bio-chemical labs and trained technicians are generally not fully available[1].
For these applications, careful consideration must be applied to the design of microfluidic system and the immobilization method of the reactive groups on the surface of the material, to ensure correct sensing function. The material used for device fabrication is also key. In microfluidics, there are various fabrication materials available, including silicon, glass, metals, ceramics, and polymers. However, for many diagnostic applications, especially in point-of-care, polymers are generally a preferred choice because they can be light, optically transparent, biocompatible, and low-cost[2].
Between the various polymeric material options, styrene-(ethylene-butylene)-styrene (SEBS) elastomers are less well-known than other materials, such as polydimethylsiloxane (PDMS) or polystyrene (PS), which are the standard. SEBS polymers, like Flexdym, have many advantages for microfluidic diagnostics and detection applications, including flexibility, robustness (i.e. sterilizable and storable), low cost, and thermostability. They can also be functionalized in a variety of manners, thus providing a wide range of opportunities to fine-tune their surface chemistry depending on the goal.
Two key aspects of the biomolecular interactions on SEBS are the binding affinity and specificity. Generally, it is also admitted that parameters such as topology, matrix surface morphology, physical structure, chemical nature, and biological composition – as well as the particular distribution on the surface – play a key role in the interactions with target biological molecules[3].
More precisely, SEBS/biomolecular interactions can be selected based on the affinity, specificity, modularity, and dynamics of the interaction. In addition, different biomolecules also give a limited window for stable experimental conditions according to temperature, pH, and osmolarity. Finally, the key features of SEBS/biomolecular interactions can be modulated by design to generate biorecognition systems with optimized properties for particular applications. The design of functionalized SEBS bio-interfaces is crucial since they are directly involved in the interaction of synthetic material with the particular biomolecule, microorganism, or cell[4].
The surface of a particular material used in biotech applications requires the control of the surface chemical composition. SEBS polymers are hydrophobic and usually inert in nature.
Researchers have therefore developed different techniques for polymer surface modification, in order to achieve polarization for the biomolecule immobilization process[4].
Different Possible Polymer Surface Functionalization Treatments
Wet Chemical Surface Modifications Wet chemical treatments refer to those approaches in which a liquid reagent is brought in contact with the surface to be treated. Typically, a strong acid or base is employed to create oxygen-containing groups. Among the acids, employed chromic acid, potassium permanganate, or mixtures of different acids (e.g., chromium trioxide-sulfuric acid) have been used to treat polypropylene (PP)[5] [6].
Self-Assembled Monolayers Self-assembled monolayers refer to the immobilization of organosilane derivatives onto inorganic substrates by chemical reaction and simultaneous formation of a self-organized silane monolayer. In this approach, the hydroxyl groups of a particular inorganic surface (i.e. glass, silicon wafers), are employed to covalently anchor chlorosilanes[7].
Surface Polymer Coatings Different approaches that were developed in the past, now provide a large range of options to deposit coatings on polymer surfaces for biomedical purposes. For instance, coatings have been deposited from the vapor phase by using laser-based processes[8], plasma and hot filament polymerization, as well as chemical vapor deposition (CVD) polymerization[9].
Photon Irradiation Photon irradiation includes the use of UV or O2 Plasma sources, either to create chemical and physical surface changes or, when the applied power increases, to induce the ablation of the material. Surface chemical modification using photons has been largely employed to produce chemical changes[10].
Ion-Beam Modification of Polymer Surfaces Ion-beam irradiations of polymers have associated different types of chemical reactions: reduction, oxidation, crosslinking, ion-implantation, loss of heteroatoms, and loss of aromaticity via ring-opening[11].
Surface Segregation of Polymeric Additives Surface segregation is a phenomenon common to all classes of materials. From the thermodynamics point of view, segregation phenomena are driven by the tendency of the system to decrease its enthalpy. For example, this can be achieved by lowering the number of unfavorable bulk contacts. In addition to polymeric melts, entropy can also serve as a significant added driving force for surface segregation[12].
Biotin-Avidin and Streptavidin-Biotin These complexes are among the most commonly utilized molecular interactions, due to their strong binding force compared to other non-covalent forces. There is a highly specific molecular recognition interaction between the two biomolecules[13].
Proposed strategies of SEBS surface polarization and covalent immobilization of bioactive molecules
SEBS, such as FlexdymTM, is a type of thermoplastic elastomer (TPE). SEBS is produced by hydrogenation of SBS, which has a high content double bond structure[14]. Because the carbon-carbon double bond of butadiene in SEBS is hydrogenated, SEBS has not only good weathering resistance, heat resistance, resistance to compressive deformation, and excellent mechanical properties, but also high rigidity and anti-aging properties. At the same time, the surface of SEBS is inert and ideal for surface functionalization such as bioactive molecule immobilization. SEBS surface polarization by -OH group is possible by chemical grafting of maleic anhydride, UV, or O2 Plasma irradiation.
The maleic anhydride grafted SEBS (SEBS g MA)
Industrialized production of maleic anhydride (MA) grafted SEBS (SEBS-g-MA)is able to increase the material’s polarity, and improve the compatibility and mechanical strength of the composites formed with inorganic materials. SEBS-g-MA is often added to polypropylene (PP) or high-density polyethylene (HDPE) to form composites, such as PP/SEBS-g-MA/inorganic material. The MA grafting method is carried out in two phases, in the presence of high temperatures. The MA grafting process occurs at 100°C and SEBS-g MA modification by ethanolamine (EA) is conducted at 135°C (Figure 2), SEBS-g-MA modified with EA possesses good compatibility, thermal stability, and mechanical properties. In the presence of imide structures with a hydroxyl group, there is further beneficial immobilization of bioactive molecules on the polymer surface[15].
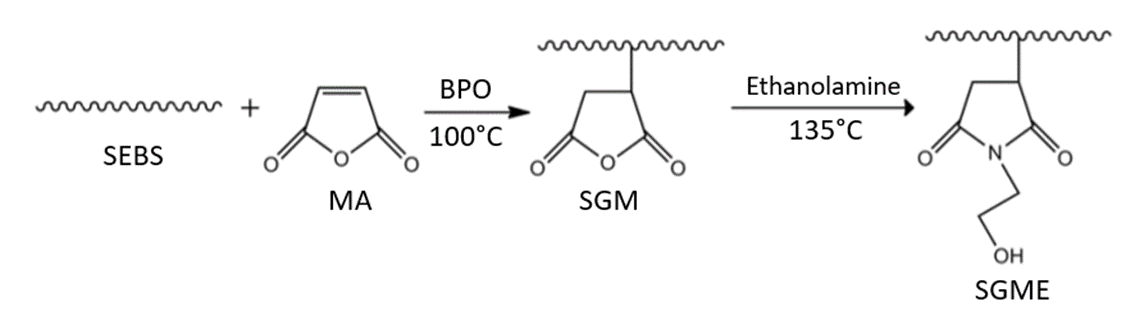
Figure 2. The reaction of MA grafted SEBS and the subsequent reaction with EA.
SEBS modification with O2 Plasma and UV irradiation
O2 Plasma discharge on SEBS polymer is a proven method to lower the hydrophobicity level of the SEBS – as can be observed with Flexdym. The incorporation of desired functional groups (-OH) onto the SEBS surface can be accomplished via this rapid method (Figure 3). The virgin SEBS film is subjected to oxygen plasma for varying time ranges, e.g. 90-120 s, and at pressures of approximately 16.5 Pa[16][17]. The resulting SEBS-OH polymer can then be treated with UV irradiation or other chemical treatments, in order to achieve bioactive molecule immobilization.
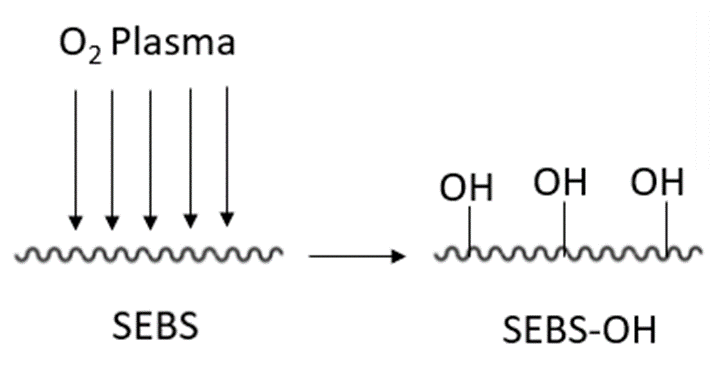
Figure 3. The reaction if O2 Plasma discharge on SEBS polymer
UV graft polymerization improves surface performance of the substrate without affecting its bulk properties. Photografting is typically conducted by bringing the substrate in contact with a solution of monomers and photoinitiator under UV irradiation. Researchers have demonstrated UV irradiation can be conducted under a high-pressure mercury lamp (400 W, main wavelength 380 nm) of Glycidyl methacrylate (GMA)[18]; using Poly(ethylene glycol) methyl ether methacrylate (PEGMA)[19] (Figure 4); or using N-vinyl pyrrolidone (NVP)[20] monomers (10 vol%) deposits. In each case, the functionalized SEBS-OH polymer is ready for chemical treatment and immobilization of targeted bioactive molecules.
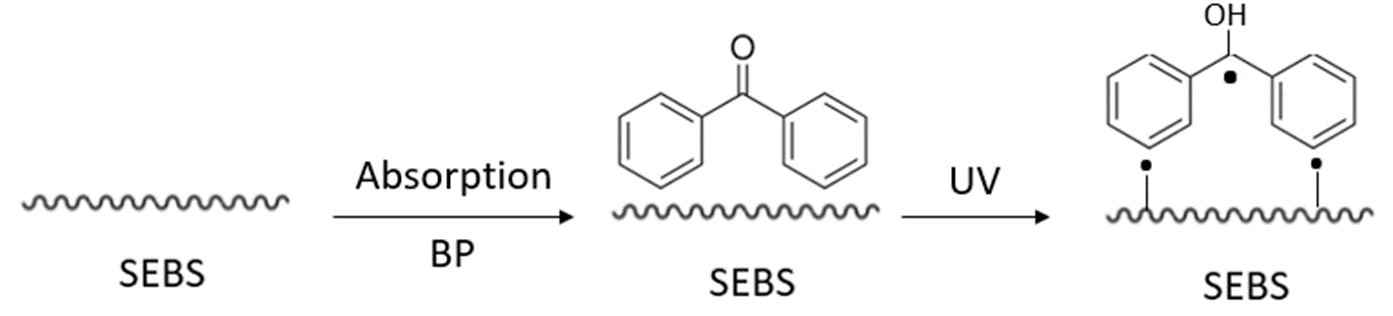
Figure 4. The reaction of UV irradiation on SEBS polymer
Bioactive molecule immobilization on SEBS-OH by Divinyl Sulfone (DVS) Method
The divinyl sulfone (DVS) method of activation works well with almost any hydroxylic or carboxylic polymer (i.e. cellulose, dextran, agarose, Sepharose, Sephacryl, Fractogel, Ultrogel, Trisacryl, and so on). It is fairly rapid (< 2 h) and the activated material is stable for up to 12 months in aqueous suspension at 4~. The reagent itself is moderately expensive but is used in small amounts, and its toxicity is limited – however avoid skin contact, or inhalation . Vinyl sulfone activated gels couple efficiently to proteins and thiols in the pH range of 6.5-10.0 and carbohydrates in the pH range of 9.5-12.5 (Figure 5). Because of the alkaline lability of the vinyl sulfone linkage, monovalent ligands (e.g., amino acids) are likely to hydrolyze from the support at pH > 8.0; however, proteins (with multivalent points of attachment) invariably show less leakage at alkaline pH[21].
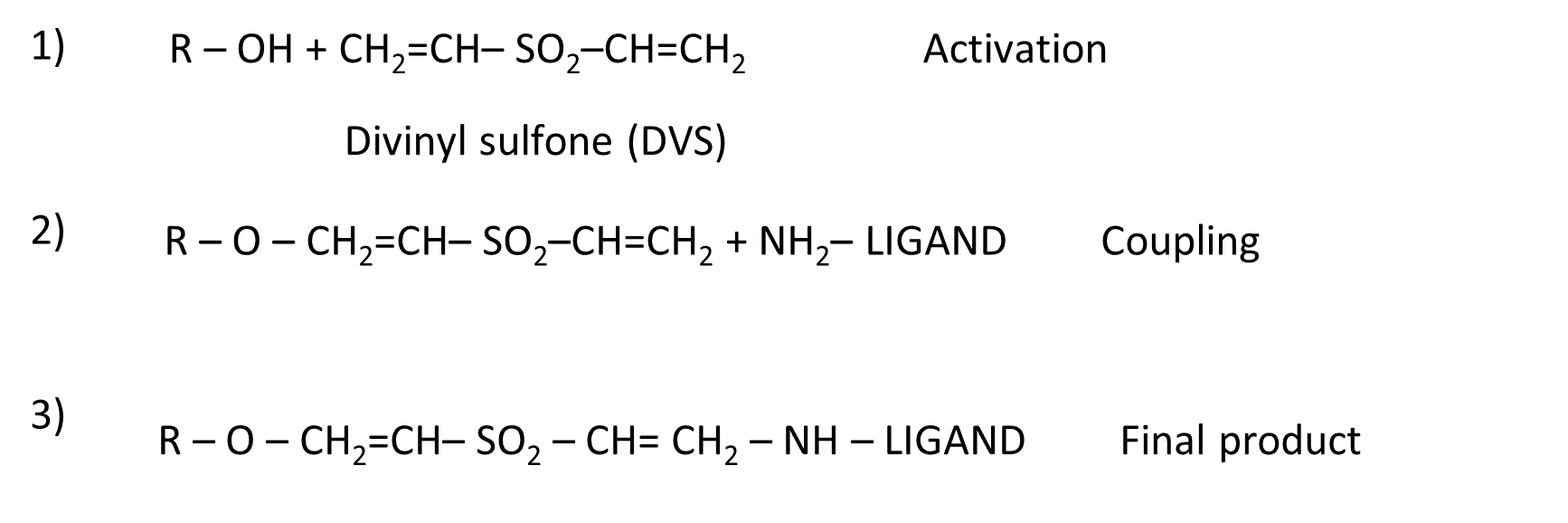
Figure 5. Chemistry of activation and coupling with divinyl sulfone
Bioactive molecule immobilization on SEBS-OH by “Schiffs” Method
Periodate activation of Sephacryl is the best example of a wide range of methods that depend on the reductive amination of a “Schiffs” base formed between an aldehyde and an amine (Figure 6). Generally, the aldehyde is formed on the solid phase and the amine is provided by a protein; however, it can also work with an amine (or hydrazide) on the gel polymer and an aldehyde provided by the sugars on a glycoprotein or polysaccharide. In reality, the individual methods differ widely in their convenience and efficiency of coupling[18].
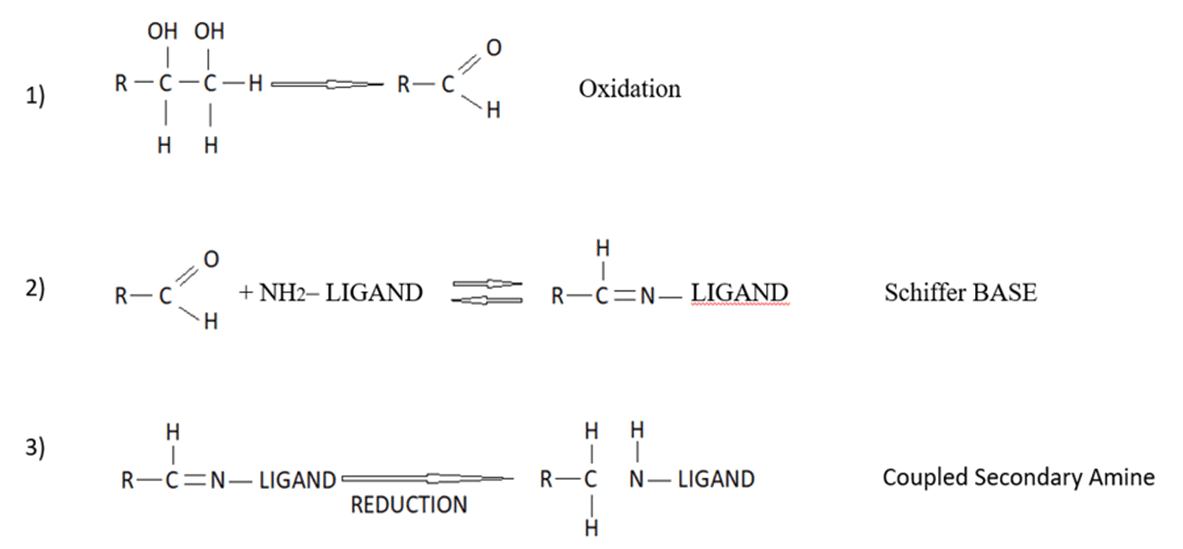
Figure 6. Periodate activation, Schiffs base formation, and reductive amination
Bioactive molecule immobilization on SEBS-OH by "BUDGIE" Method
This method involves converting epoxy groups to diol (also aldehyde), carboxyl, sulfopropyl, amino, hydrazino, IDA (metal chelate), and DEAE groups, as well as others (Figure 7). Additional advantages of epoxy activation with the “BUDGIE” reagent include its low leakage ether bond and flexible extended hydrophilic spacer arm. However, one must consider the disadvantages of high pH activation, slower reactivity, and the inconvenience and inefficiencies of subsequent step derivatizations [18].
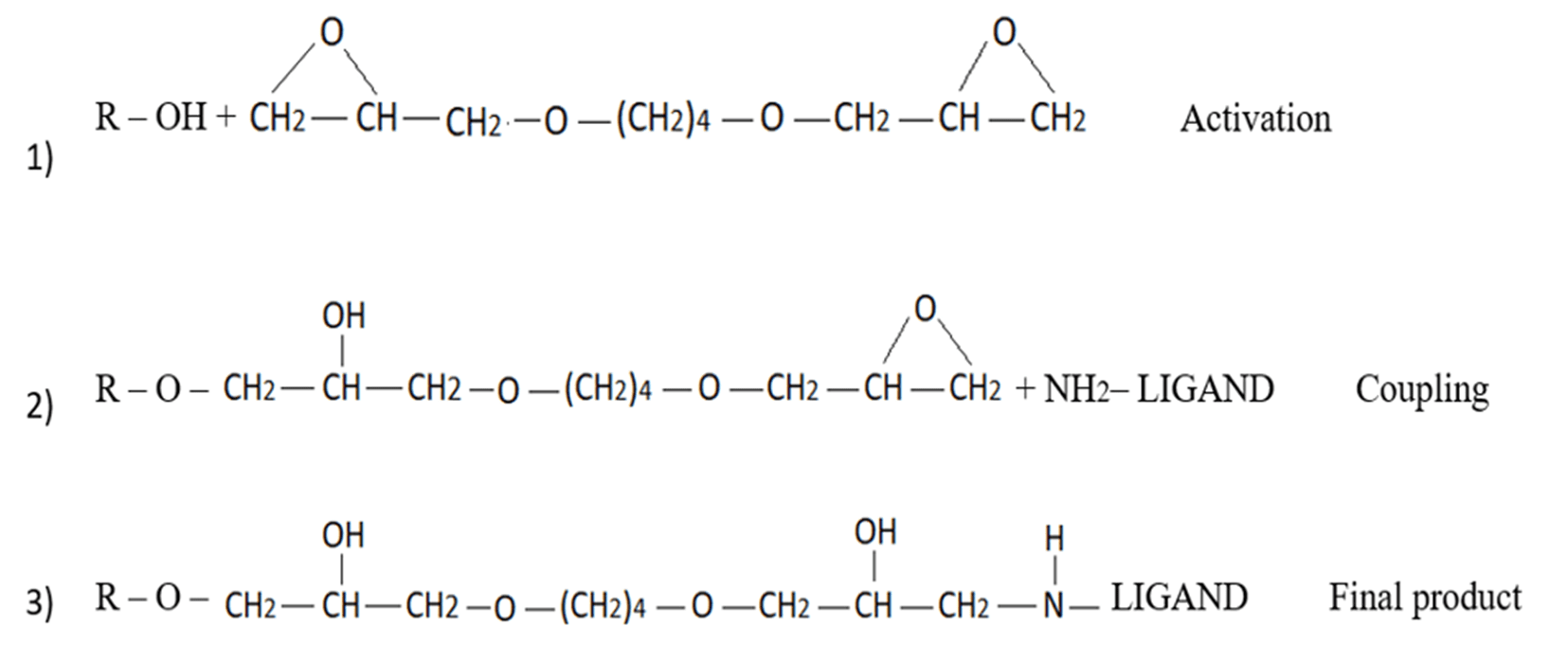
Figure 7. Chemistry of bis-epoxide (“BUDGIE”) activation and coupling
Bioactive molecule immobilization on SEBS-OH via the glutaraldehyde Method
Glutaraldehyde (GA, pentane-1,5-dial) is a dialdehyde that can react with four hydroxyl groups of SEBS-OH, and can therefore be used as a crosslinking agent to modify the SEBS-OH surface. Each aldehyde can react with one hydroxyl group to form a hemiacetal (Figure 8a.), and subsequently with a second to form a full acetal (Fig. 8b). Whereas the former group is susceptible to hydrolysis, the latter is stable under both neutral and acid conditions[22].
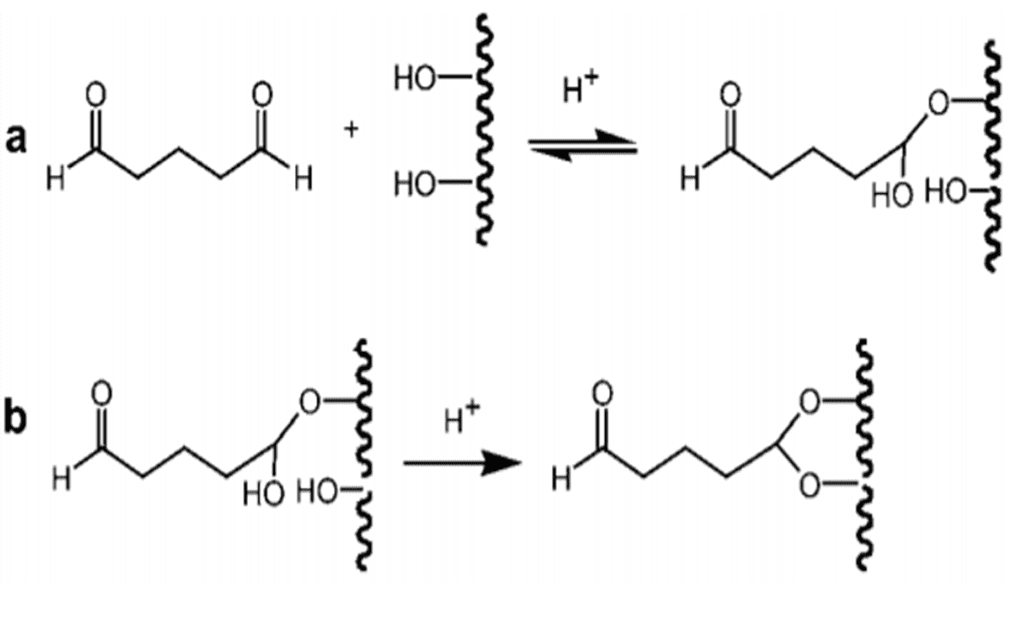
Figure 8. The reaction of glutaraldehyde with one hydroxyl group forming a hemiacetal (a) and subsequently with another hydroxyl group forming a full acetal (b)
The degree and quality of immobilization can be estimated via different methods: by UV absorbance, where the difference is measured before/ after coupling; by BCA protein assay directly on the SEBS material; by Coomassie dye uptake indirectly, where the difference before/after mixing SEBS and the dye is measured; or by labeling of proteins by fluorescence reagents18.
Assuming the activation and coupling are both performed successfully, it is important to evaluate the functional performance (i.e. specific biological activity U/g of the polymer) of the protein. In general, enzymes and antibodies have well-known implemented assays. However, other group-specific or nonspecific ligands also need to be tested for binding capacity to their target. It should not be assumed that efficient coupling will always lead to a biologically functional ligand. The residual concentration of targeted ligands should be followed by Nanodrop fast method. Here, all targeted biomolecules could be stained by fluorescence staining kit (e.g.Tracy 645 Protein labeling kit) and the fluorescent proteins detected by fluorescence microscope on the ESBE surface[18].
Surface Functionalization : Conclusion
The covered SEBS-OH chemical treatment methods for biomolecule immobilization can be operated with the same mechanical equipment and no one item is essential, but it is worth trying to use them all as it makes a far simpler and more reliable system.
Here, we have described approaches that are simple, reliable, cheap, convenient, and can be used in most cases by researchers who are not direct trained chemists. Several principles underlie the choice of methods, namely avoidance of organic/anhydrous solvents, ability to easily scale up or scale down quantities, single-step reactions, ease of quantitation of product, activated SEBS should be stable in aqueous suspension at 4°C for several months, unpleasant or toxic reagents are avoided, and last but not least, the efficiency of coupling of valuable bioactive molecules should exceed at list 90%.
References
[1] Fong Lei et al. Design of Polymeric Platforms for Selective Biorecognition (2015), DOI 10.1007/978-3-319-17061-9_11
[2] Delaittre. Design of Polymeric Platforms for Selective Biorecognition (2015), DOI 10.1007/978-3-319-17061-9_4
[3] Rodríguez-Hernández et al. Design of Polymeric Platforms for Selective Biorecognition (2015), DOI 10.1007/978-3-319-17061-9_1
[4] Rodríguez-Hernández. Design of Polymeric Platforms for Selective Biorecognition (2015), DOI 10.1007/978-3-319-17061-9_2
[5] Tao et al. Macromolecules (2001), DOI.org/10.1021/ma010941b
[6] Sheng et al. Journal of Adhesion Science and Technology (1995), DOI.org/10.1163/156856195X00284
[7] Ulman. Chemical Reviews (1996), DOI.org/10.1021/cr9502357
[8] Chrisey et al. Chemical Reviews (2003), DOI: 10.1021/cr010428w
[9] Schiller et al. Chemistry of Materials (2002), DOI.org/10.1021/cm011139r
[10] Chtaib et al. Journal of Vacuum Science & Technology aVacuum Surfaces and Films (1989), DOI: 10.1116/1.576341
[11] Pethrick. Carl Hanser Verlag, Munich, Vienna, New York, 1993.
[12] Walton & Mayes. Physical Review E (1996), DOI: 10.1103/PhysRevE.54.2811
[13] Hyun et al. Nano Letters (2002), DOI: 10.1021/nl0257364
[14] Ruzette &Leibler. Nature Mater (2005), DOI.org/10.1038/nmat1295
[15] Chuang & Nien. Journal of Polymer Research (2019), DOI.org/10.1007/s10965-019-1723-7
[16] Hou et al. Colloids and Surfaces B: Biointerfaces (2013), DOI.org/10.1016/j.colsurfb.2013.06.029
[17] Luan et al. Colloids and Surfaces B: Biointerfaces (2012), DOI:10.1016/j.colsurfb.2011.12.027
[18] Xin et al. Journal of Biomaterials Science Polymer Edition (2014),
[19] Li et al. Applied surface science. (2012), DOI.org/10.1080/09205063.2014.918458
[20] Luan et al. Colloids and surfaces B: Biointerfaces (2012), DOI.org/10.1016/j.apsusc.2011.10.024
[21] Pepper. Molecular Biotechnology (1994), DOI: 1073-6085/1994/2:2/157-178/58.40
[22] Xu et al. J. Appl. Polym. Sci (2004), DOI.org/10.1002/app.20593
The Author
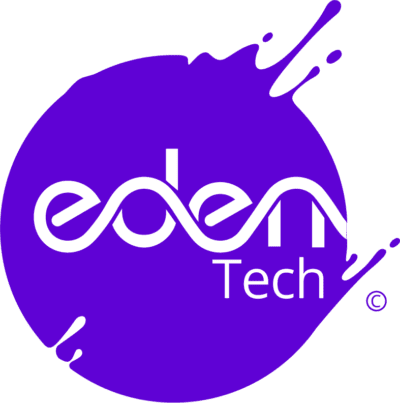
George Songulashvili, PhD
Eden Medtech R&D