Microfluidic Mixing: How to Design Efficient Micromixers for Lab-on-a-Chip Devices
Microfluidic mixing is at the core of high-performance lab-on-a-chip systems. From PCR preparation to chemical synthesis and diagnostics, precise and fast mixing at the microscale is essential. But in microfluidics, where laminar flow dominates, achieving effective mixing is notoriously difficult.
This guide provides design principles, best practices, and real-world applications to help engineers and researchers build efficient micromixers, whether passive or active. If you’re designing a microfluidic device, understanding mixing mechanisms is crucial to ensure high performance, reproducibility, and time savings.
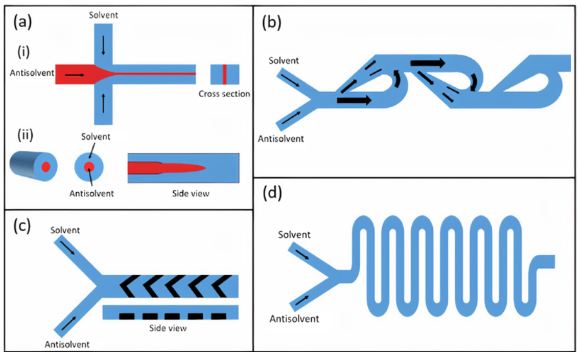
Why Is Microfluidic Mixing So Challenging?
In microfluidic systems, the Reynolds number is typically less than 1, meaning flows are laminar, with no turbulence. As a result, diffusion is the dominant mixing mechanism, slow, especially in narrow channels.
Poor mixing can negatively impact:
-
Reaction kinetics in chemical and biological assays
-
Sample preparation times
-
Reagent homogeneity in diagnostics
-
Accuracy in single-cell or PCR workflows
That’s why choosing the right micromixer design is vital for success.
Passive vs. Active Microfluidic Mixing: Which One Should You Use?
Passive Micromixing
Passive micromixers rely on channel geometry to improve mixing without any external energy. By stretching, folding, or splitting flow paths, they enhance diffusion through clever design alone.
Common passive micromixer designs:
- T-shaped or Y-shaped junctions
- Herringbone structures (e.g., Staggered Herringbone Mixer, SHM)
- Serpentine and spiral channels
- Split-and-recombine (SAR) mixers
These designs are available and easy to simulate using tools like FLUI’DEVICE.
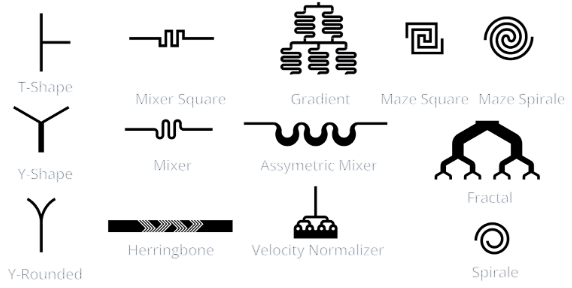
Type of passive micromixers you can find on FLUI’DEVICE
Advantages:
-
No external components required
-
Easy and cost-effective to fabricate
-
Ideal for point-of-care and low-power applications
Limitations:
-
Mixing depends heavily on flow rate and channel length
-
Less tunable or controllable during operation
Active Micromixing:
Active micromixers introduce external energy to enhance mixing using actuation mechanisms.
Common Active Mixing Techniques:
-
Electrokinetic mixing (e.g., AC electroosmosis)
-
Acoustic mixing (surface acoustic waves)
-
Magnetic bead stirring
-
Localized thermal gradients
Advantages:
-
Faster and more uniform mixing
-
Greater control over mixing dynamics
Limitations:
- More complex to design and fabricate
- Require external power or control components
How to Design a Microfluidic Mixer: Step-by-Step Guidelines
Whether you’re building a passive or active micromixer, the following design considerations are essential for success:
1. Understand Your Flow Regime
Estimate your Reynolds number. If Re < 10, diffusion dominates. Use long channels or SHM patterns. For slightly higher Re, consider vortex-inducing geometries.
2. Match Mixing Strategy to the Application
-
Passive Mixing: Ideal for diagnostics, low-cost testing kits, or simple reagent mixing.
-
Active Mixing: Best for drug delivery systems, synthetic biology, or complex chemical workflows.
3. Use Simulation Tools Before Fabrication
Simulating your mixer saves time and avoids trial-and-error. Tools like FLUI’DEVICE help you:
-
Design geometries visually
-
Simulate mixing performance
-
Predict pressure drops and flow paths
Real-World Applications of Microfluidic Mixing
Micromixers power a wide range of applications in microfluidics and lab-on-a-chip systems:
Application | Role of Mixing |
---|---|
PCR and DNA amplification | Mix primers and samples uniformly |
Drug screening | Combine reagents and cell suspensions |
Chemical synthesis | Control reaction rates and product yield |
Single-cell analysis | Precise lysis or reagent delivery |
Isothermal amplification | Improve speed and reliability of diagnostics |
Summary: Mastering Microfluidic Mixing Design
To optimize your microfluidic mixing strategy:
-
Choose the right mixer type (passive vs active)
-
Design geometries that promote efficient advection or diffusion
-
Simulate and iterate using tools like FLUI’DEVICE
-
Align the mixer’s performance with the needs of your end-use application
As microfluidics reshapes biotech, diagnostics, and drug discovery, mastering microfluidic mixing will remain a key differentiator in your lab-on-a-chip success.
Start Designing Your Micromixer Now
Try FLUI’DEVICE — a powerful browser-based tool to prototype, simulate, and export microfluidic mixer designs in minutes. Whether you’re developing passive or active mixers, FLUI’DEVICE offers:
-
Drag-and-drop interface
-
Instant simulations
-
Export options in SVG, STL, or DXF