The human digestive system is a powerful machine able to transform organic matter into energy, all while filtering out any threatening and unnecessary elements. This system is however not infallible; malfunctions can occur and pathogens can sometimes get past the screening process. To investigate these events and find a treatment or cure, researchers and pharmaceutical companies employ in vitro and animal models to first test their theories. Unfortunately, these models often fail to compare with human physiology, and conclusive results cannot be achieved. As a result, there is an urgent need to develop new more relevant models. Among these novels in vitro models, Organ-On-Chip technology is of particular interest. In this short review, we discuss how the most recent advances of this fascinating technology and how it applies to the study of the human digestive system.
Organ-on-Chips recapitulate the Digestive System
Many different types of diseases affect the human digestive system, such as Inflammatory bowel disease and ulcers in the gastrointestinal tract, hepatitis in the liver, and chronic kidney disease, to cite a few. Additionally , cancer is a constant threat to all organs involved. Therefore, pharmaceutical companies and clinical facilities are devoted to treat and cure these ailments. But to do so, they had to use animals as targets to investigate the disease progression and response to drugs. Recently, a new generation of in vitro systems has raised interest beyond the academic circle and into the industrial world, which could potentially rival the existing animal models and maybe one day replace animal testing altogether. This new system, known as Organ-On-Chip, relies on microfluidic technology (the study of liquids at the micrometer scale) to reproduce the complex mechanical and biological functions of human organs.
There are many organs involved in the functioning of the digestive system, divided into two main categories: hollow and solid. As a result, Organ-on-chips have already been developed to study each of them individually. We list below some of these organ-on-chip systems, and what a researcher has to consider when preparing such a model.
The gut-on-a-chip: First line of defense
Gut-on-a-chip platforms
The first element to consider is the interface between the epithelial and endothelial layers. The most common approach in microfluidics is to separate two channels, one apical and one basolateral, is by a porous membrane. Cells are cultured on both sides of the membrane, and an exchange of elements is observed at their interface (1). Sung et al. went a step further and developed a 3D collagen scaffold to replace the flat porous membrane (2). This scaffold made from Collagen was covered with Caco-2 cell lines and closely mimicked the colon villi structure. Comparison with traditional 2D culture first indicated a significant variation in gene expression toward in vivo like physiology (3). Moreover, when exposed to a common bacterial infection (salmonella, or S. Typhimurium), the 3D in vitro villi provided increased protection from the infection.
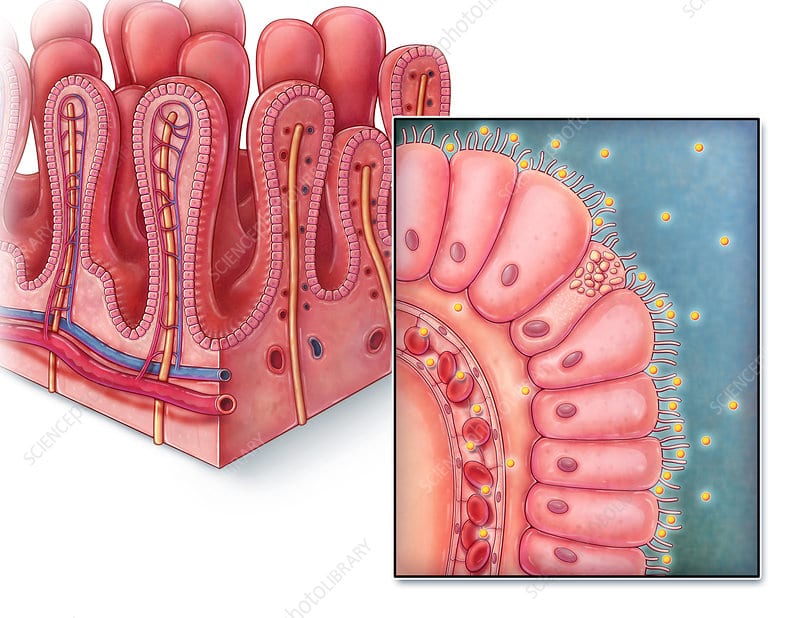
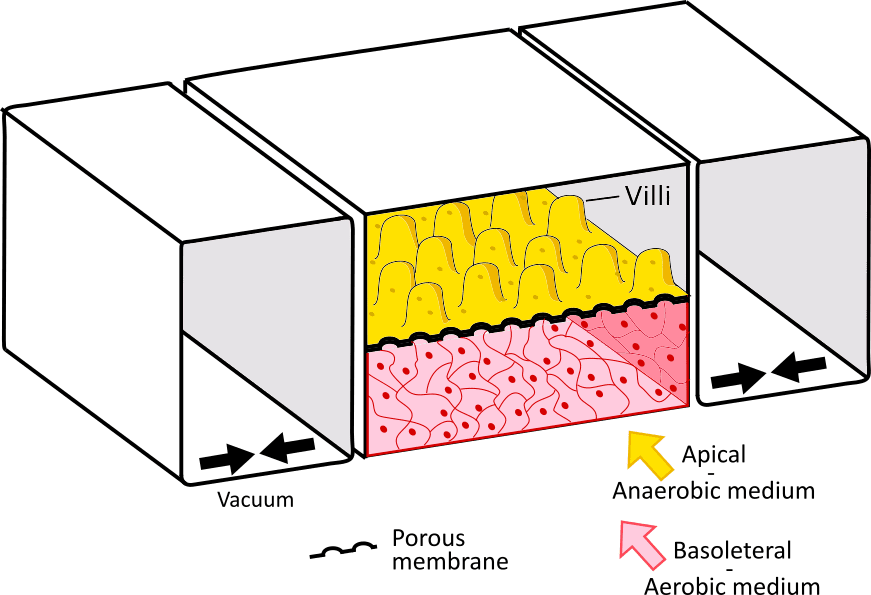
Figure 1. The Gut on a Chip, replicating the gastrointestinal wall:
On the left, Illustration of the villi structure on the gastrointestinal wall, with an epithelial layer and a network of blood vessels (image from sciencephoto.com). On the right, a gut on a chip platform composed of an epithelial layer with villi-like structure, and an endothelial layer. The two layers are separated by a porous membrane and are contained between vacuum chambers to reproduce the gut stretching motion.Β
The second element is the anaerobic condition of the intestinal tract. Recently, S. Jalili-Firoozinezhad et al. integrated oxygen gradients in the system, effectively creating hypoxic regions in the epithelium layer (4). Under these conditions, the gut-on-chip showed increased intestinal barrier function and was able to sustain a high level of microbial diversity. These bacteria included anaerobic bacteria and were present in a balanced ratio comparable to the ones found in human feces (Figure 1 right).
Finally, the last element is mechanical stimulation. It has long been determined that shear stress and mechanical actuation are major actors in cell differentiation – and gut cells are no exception. In the gut-on-chip, this translates to the periodic stretching of the membrane, mimicking the smooth muscle cell-controlled peristalsis. Kim et al. used this approach on a 2D membrane layered with human Caco-2 cells (5,6). Upon stretching the membrane (with the help of vacuum channels placed on each side of the membrane) they successfully performed cell differentiation, which in turn resulted in the spontaneous formation of villi (Figure 1 right).
Although the gastrointestinal tract can efficiently break down nutrients into smaller elements, it is insufficient to convert them into useful chemicals. The main organs responsible for this task are present outside the GI tract and are the liver, pancreas, and kidney. These organs have been extensively investigated in microfluidics and interesting Organ-on-Chips have been developed.
Liver-on-a-chip: an in vitro filtration system
The liver plays a predominant role in the maintenance of normal physiological functions, by filtering toxic elements from the digestive tract, but also the entire body. Its strength relies on its ability to repair itself. However, its efficacy can be compromised after exposure to toxins, but also to ingested/injected drugs. Traditional in vitro 2D models, as well as animal models with very different metabolism, cannot reproduce accurately the impact of drugs on the liver. A more interesting alternative solution presents itself, the liver-on-a-chip (9).
The liver is the largest solid organ in the human body, with many different cell types involved. However, its complex structure is divided into thousands of repetitive units of liver lobules, with sizes smaller than 2 mm each, which is perfect for microfluidics. Each lobule presents a hexagonal structure centered around a central vein, with a hepatic artery, bile ducts, and portal veins on the outside (figure 2 left). Lobules are mainly composed of parenchymal cells (hepatocytes) responsible for the filtration of blood sugar, decomposition of ammonia, and synthesis of bile acids, as well as drug metabolism. Other cells present include hepatic stellate (lipocytes) responsible for the prevention of fibrosis, hepatic endothelial sinusoidal endothelial cells, and the Kupffer cells, macrophages responsible for the removal of foreign elements.
Liver on a chip platforms
As in any biological experiment, the first step to develop an Organ-on-Chip is to carefully select the cells which are going to be integrated into the system. The main challenge relies upon balancing the relevance and durability of the model. On one hand, primary hepatocytes are the most relevant cells as they directly correspond to the target cells, but they can easily lose their specificity in a long-term experiment. On the other hand, hepatic-derived cell lines (e.g., HepG2) have a long life span but have shown inaccurate reactions to drugs. A common 3D in vitro model, the 3D spheroid culture, an aggregate of cells of one or multiple origins, has already been shown to easily alleviate this issue. Several studies successfully cultured spheroids of primary hepatocytes for up to 9 days without losing functionality under static perfusion conditions (9, 10).
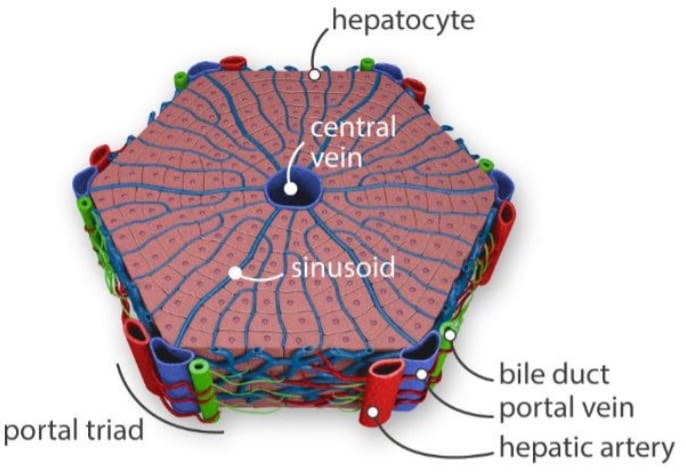
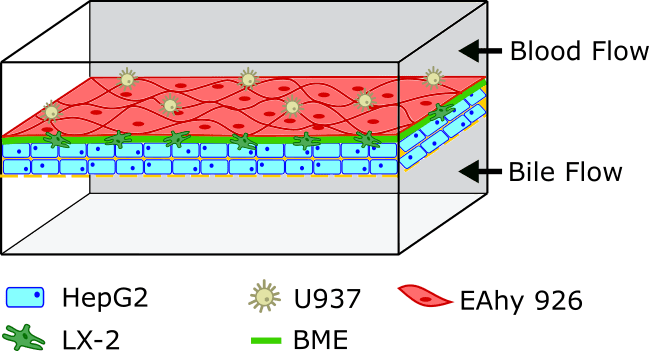
Figure 2. The liver on a chip filters the toxins.
On the left, illustration of a liver lobule, basic block of the liver, filtrating toxins from the ventral vein to the portal triad(bile duct, portal vein, and hepatic artery) (8). On the right, Liver-on-a-chip with a layer-by-layer approach; cells of different composition are subsequently deposited on a porous membrane, recreating the lobule complex structure. The two channel then represent the central vein and he bile duct.
If the 3D spheroid model is an easy method to implement, it is sometimes insufficient to translate the necessary information. An alternative option is a matrix-dependent structure. In it, the microfluidic device developed presents a unique architecture, which allows the aggregation of the hepatocytes in a dedicated compartment, embedded in a hydrogel matrix (e.g., collagen). Under this condition, it becomes possible to continuously perfuse the cells, adding a mechanical actuation (i.e., shear stress) preventing the de-differentiation. Moreover, this matrix approach leaves room for the cells to rearrange themselves, and more easily communicate or access necessary nutrients.
Going one step further, it has been shown that the selection of the hydrogel in the matrix-dependent structure could limit the relevance of the model, as it often doesnβt correspond to the natural hepatic collagen composition. Instead, a layer-by-layer deposition approach has been proposed (Figure 2 right). Not unlike the model presented in the gut-on-chip model, the microfluidic device is designed as two channels separated by a porous membrane. The top channel represents the central vein, and the bottom the bile duct. In the top channel, cells are deposited on the membrane layer by layer first hepatic, then endothelial. By using this approach, Kang et al. were able to maintain the morphology of the hepatocytes for up to 30 days, all the while converting ammonia into urea (12).
Pancreas-on-a-chip: Diabetes treatment quality control
The Pancreas plays two important functions in the human body. The first relates to its role in the digestive system, as it secretes enzymes into the duodenum (small intestine) to help break down proteins and other elements. Its second role, although just as important, is investigated with much more scrutiny, as it involves the secretion of insulin and the regulation of glucose in the bloodstream. Indeed in 2018, according to the CDC, approximately 1.5 million new cases of type II diabetes (insulin-dependent) were identified in the US alone (13). This is an alarming number that has kept increasing in the last fifty years. Moreover, this disease has currently no known cure, and commercialized treatments require a daily dose of insulin injection. In this particular instance research in the field of pancreas-on-chip not only proposes interesting models to investigate the disease but also assists in the development of high-quality treatments.
pancreas-on-a-chip systems
Type II diabetes occurs usually as a result of obesity and a lack of exercise. Concretely, cells become resistant to the action of the insulin produced by the beta cells present in the islets of Langerhans, inside the pancreas. In turn, the amount of insulin secreted becomes insufficient to efficiently down-regulate the glucose concentration in the bloodstream. The affected patient becomes susceptible to cardiovascular diseases, as well as many other major illnesses (e.g., kidney failure, dementia). Besides the daily dose of insulin injection to compensate for insulin resistance, a major approach of long-term treatment is the injection of pancreatic islets, which will in turn continuously secrete the required hormone. The two main challenges of this approach are to manage the long-term viability and function of the injected islets and ensure their immune compatibility with the body.
Several methods have been developed to investigate the efficacy (glucose-induced insulin secretion) of isolated healthy islets before integration, with the help of microfluidics (14). The main approach consists of measuring the intracellular calcium (Ca2+) concentration variation. Lomasney et al. used this approach to observe with precision the response time, in terms of insulin production level, as a response to various concentrations of glucose (15).
A second parameter to assess the quality of the islets is their oxygen consumption rate. Islets are aerobic cells, and healthy islets consume more oxygen than compromised ones. With the help of microfluidics, it has been made possible to measure accurately the oxygen consumption of isolated islets. Interestingly, Β in E. Taddeo and colleaguesβ recent study, not only were they able to confirm the impact of oxygen on theΒ insulin secretion rate of islets stimulated by glucose; they also observed a variation in insulin production between islets of the same pancreas (16). This information reinforces the idea of a quality control tailored to a single islet level, rather than only a healthy pancreas donor.
Kidney-on-a-chip: Waste disposal system
The kidney, the last organ on our list, plays many roles as a regulation system. In the digestive system, it is responsible for filtering and removal of waste products and drugs from the blood. It also secretes vitamin D for the bones and hormones for the stimulation of red blood cell production by the bone marrow. Several factors, including age, high blood pressure, and diabetes, can affect the ability of the kidneys to filter out waste from the body. In turn, the waste accumulated in the body can cause a multitude of severe symptoms and can be fatal if untreated. Kidney failure accounts for 8-16% of deaths worldwide and is a very expensive disease to treat (18).
The main function of a kidney is to filter the blood and is designed to maximize the volume of blood treated per unit of time. To achieve this, its architecture divides the entry vein (renal arteries) into series of small capillary vessels. The capillary network (glomerulus) is divided into nephrons, the functional unit of the kidney (Figure3 left). In each nephron, filtration of the blood is performed by filtrating the waste through the glomerular filtration barrier on the blood vessel wall. Thanks to microfluidics, it is possible to recreate this glomerular barrier with the kidney-on-a-chip technology, to investigate barrier degradation and response to drugs (19).
Kidney-on-a-chip systems
Once again, the most common kidney-on-a chip design consists of two channels separated by a porous structure or membrane. The first channel represents the glomerulus and is loaded with glomerular endothelial cells. The second channel is layered with podocytes, representing the bowmanβs capsule, where the waste is collected in the urine. Using a complex microfluidic device incorporating Matrigel as support, Wang et al. were able to recreate the interface glomerulus/capsule (19). With their platform, they were able to successfully recreate diabetic nephropathy, a phenomenon previously unobservable in either in vitro or animal models.
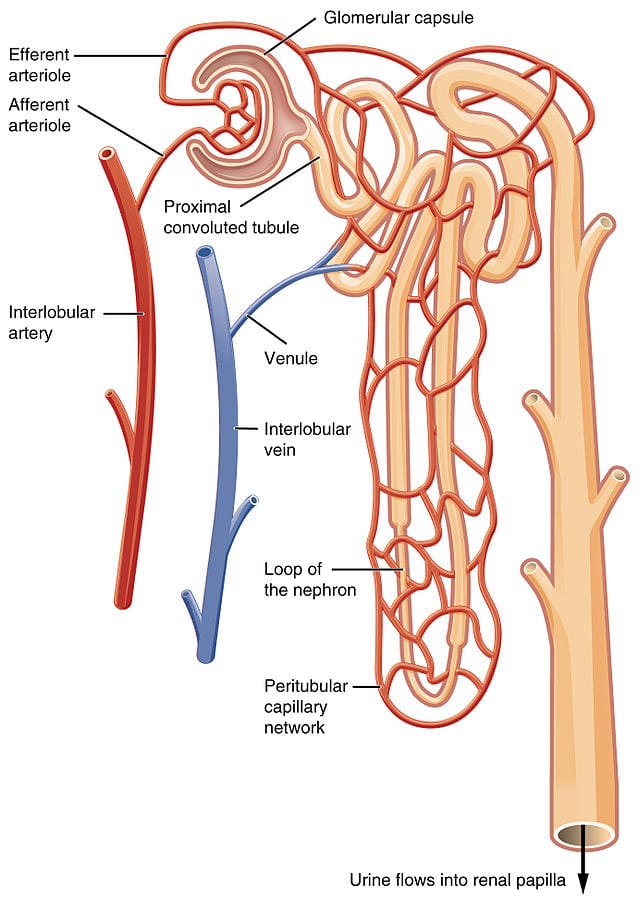
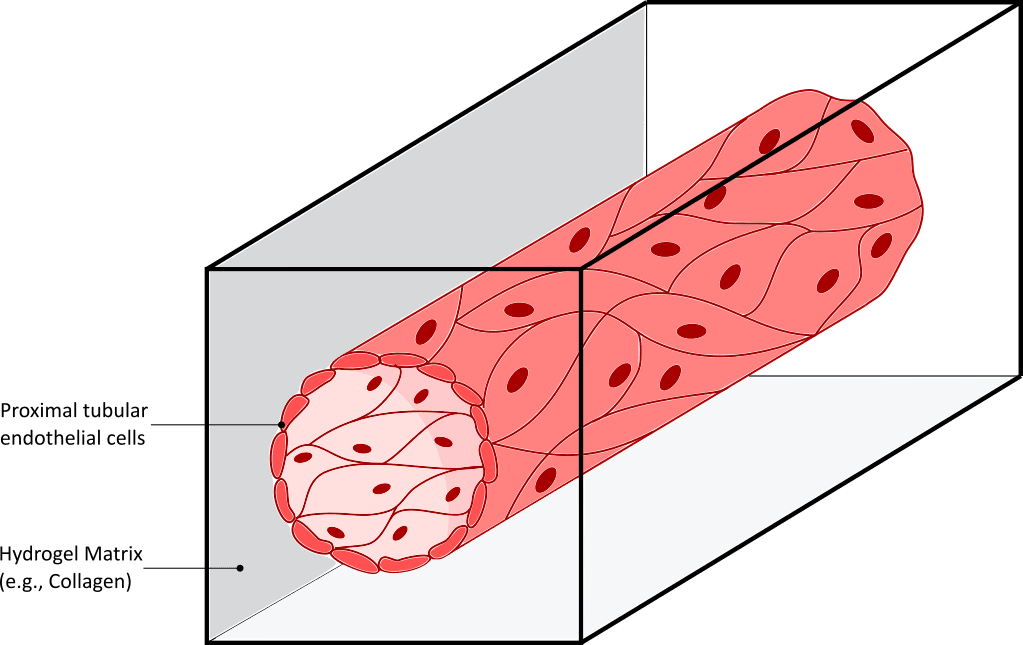
Figure 3. The kidney-on-a-chip recreates diabetic nephropathy.
On the left Illustration of the functional unit of the kidney: the nephron. On the right, a proximal tubule on-a-chip, prepared by injectingΒ proximal tubular endothelial cells on a hollow lumen of hydrogel, recapitulates cell morphology, polarity, and kidney cilia sprouting.
Two other sections of the nephron: the proximal tubule and the distal tubule (situated before and after the glomerulus respectively), are also important elements of the kidney function, as they help regulate concentrations of potassium, sodium, calcium, and pH. These sections have also been developed using organ-on-chip technology. Several proximal tubules have successfully been reproduced, by injecting proximal tubular endothelial cells (hPTECs) inside a hollow lumen prepared from hydrogel (fibrin or collagen-based) (Figure 3 right). Interestingly, cells in this cylindrical organ-on-a-chip conformation maintained viability and differentiation level for a longer time, compared to cultures in 2D (20). More importantly, the homogeneous distribution of shear stress on the cells induced cell morphology and polarity changes toward in vivo like structure. Primary kidney cilia, whose purpose is currently highly investigated, have also been shown to reappear as a result of shear stress.Β
Consideration and future Challenges
As described in this overview, organ-on-chip technology is a powerful approach to the development of in vitro models with relevant physiological and even mechanical functions. These setups however remain limited as compared to the in vivo conditions. Despite that, they continue to bring remarkable insight into our previously misunderstood pathologies. They also assist in the development and test of new treatments, for the most challenging diseases. And they surely have the potential to do more.
While each organ-on-a-chip is a limited representation of the organ it mimics, it can be used in connection with other organ-on-chip systems. Just like the human digestive system relies on the complete functioning of all its organs involved, it is possible to develop a complex network of microfluidic systems reproducing the complete path of the nutrients/drugs from one organ to the next. With such systems, it would become possible to ascertain in vitro the effects and side-effects of a drug after its ingestion. Going one step further, the human body-on-a-chip is an ambitious project first proposed by the Wyss Institute, aiming to connect all the organs of the human body. This setup would not only reproduce the function of each organ, but also the communication between them. Several ventures like ORCHID have begun researching solutions to this incredible challenge. Even more interesting, it would be possible to prepare all organs from a single donor, effectively turning the system into a point-of-care device (POC) with unprecedented features and relevance for personalized care.
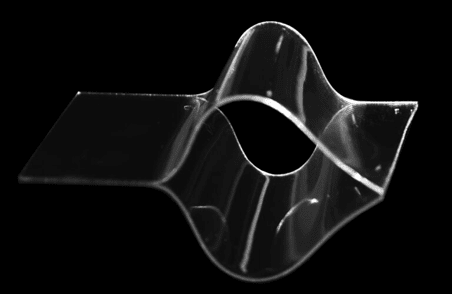
FledymTM Polymer is aΒ biocompatible, cheap, flexible, and easy-to-handle material ideal to prepareΒ yourΒ microfluidic devices.
Finally, most organ-on-a-chip systems are either prepared from plastics or a transparent polymer known as PDMS (polydimethylsiloxane). Plastic is usually the cheapest option but requires access to advanced machinery, and is not profitable or even practical at the proof-of-concept or prototype level. On the other hand, PDMS is an easy-to-handle material that requires little equipment and can be prepared anywhere. Its main limitations reside in the lack of high reproducibility in the material structure; its oxygen permeation; its difficult scalability and assembly for production; and its tendency to absorb chemicals. For these reasons, pharmaceutical companies prefer to utilize thermoplastic materials, which involve complex and sometimes expensive prototyping. To help solve this issue on the material selection, Eden Tech has developed a new thermoplastic elastomer material, FlexdymTM, which brings together the benefits of both thermoplastics and elastomers, like PDMS. Β A Transparent material, Flexdym is a biocompatible, cheap, flexible, and easy-to-handle polymer, which can be prepared using a simple protocol and affordable equipment. To further assist academic research and companies alike, Eden Tech provides a series of equipment to reproduce devices with high fidelity and reproducibility, with minimum training required.
References
- Y. Xiang , 2020, Gut-on-chip: Recreating human intestine in vitro π
- J.H. Sung et al. , Microscale 3-D hydrogel scaffold for biomimetic gastrointestinal (GI) tract model, Lab Chip, 2011, 11, 389 π
- S.H. Kim, Three-dimensional intestinal villi epithelium enhances protection of human intestinal cells from bacterial infection by inducing mucin expression, 2014 π
- S. Jalili-Firoozinezhad, A complex human gut microbiome cultured in an anaerobic intestine-on-a-chip, 2019 π
- H.J. Kim et al., Human gut-on-a-chip inhabited by microbial flora that experiences intestinal peristalsis-like motions and flow, 2012 π
- H.J. Kim et al., Co-culture of Living Microbiome with Microengineered Human Intestinal Villi in a Gut-on-a-Chip Microfluidic Device, 2016 π
- C. Costello et al.,3βD Intestinal Scaffolds for Evaluating the Therapeutic Potential of Probiotics, 2015 π
- Leo A. van Grunsven, 3D in vitro models of liver fibrosis, 2017
- J. Deng, Engineered Liver-On-A-Chip Platform to Mimic Liver Functions and Its Biomedical Applications: A Review, 2019 π
- A. Aeby, Microfluidic Hydrogel Hanging-Drop Network for Long-Term Culturing of 3D Microtissues and Simultaneous High-Resolution Imaging, 2018 π
- Y. Toh et al., A microfluidic 3D hepatocyte chip for drug toxicity testing, Lab Chip 2009 π
- Y. Kang et al., Metabolic Patterning on a Chip: Towards in vitro Liver Zonation of Primary Rat and Human Hepatocytes. Sci. Rep. UK 2018 π
- CDC National Diabetes statistic report 2020Β
- S. Abadpour et al., Pancreas-on-a-Chip Technology for Transplantation Applications, 2020 π
- A. Glieberman et al., Synchronized stimulation and continuous insulin sensing in a microfluidic human Islet on a Chip designed for scalable manufacturing, 2019 π
- A. Lomasney et al., Simultaneous Monitoring of Insulin and Islet Amyloid Polypeptide Secretion from Islets of Langerhans on a Microfluidic Device, 2013 π
- E. Tadeo et al., Individual islet respirometry reveals functional diversity within the islet population of mice and human donors, 2018 π
- J. Wu et al., Lab-on-chip technology for chronic disease diagnosis, 2018 π
- N. Ashammakhi et al., Kidney-on-a-chip: untapped opportunities, 2018 π
- L. Wang et al., A disease model of diabetic nephropathy in a glomerulus-on-a-chip microdevice, 2017 π
The Author :

Dr. Jean-Baptiste BlondΓ©
Eden Tech Microfluidic Simulation and Web Expert
Ph.D. in Biomedical Engineering for the University of Twente, The Netherlands