The Potential of 3D Printing using 2PP technology in Skeletal Muscle Engineering - Application Note
Introduction
3D printing has revolutionized various fields by enabling precise and customizable fabrication of complex structures. In the context of skeletal muscle engineering, 2-photon polymerization (2PP)-based 3D printing offers significant potential to overcome existing limitations in scaffold and microfluidic design. This application note explores the advantages, methods, and applications of 2PP-based 3D printing for skeletal muscle engineering based on the results from a PhD thesis.
Skeletal Muscle Tissue Engineering: the synergistic combination of hydrogel-based scaffolds and microfluidic systems
Skeletal muscle tissue engineering has advanced significantly in recent years, with the emergence of increasingly sophisticated in vitro models, which aim to recapitulate key features of native tissue, including:
- Alignment and structure of muscle cells
- Co-culture of different cell types present in the muscle niche
- Provision of mechanical and electrical stimuli
Microfluidic devices have emerged as promising tools for modeling the skeletal muscle niche, due to their ability to:
- Create fluidic compartments that support co-cultures
- Precisely control concentration gradients through dynamic flow conditions
- Provide mechanical and electrical stimuli to cells in culture
However, traditional microfluidic devices have limitations in terms of tissue size and accessibility. Scaffold-based in vitro skeletal muscle models offer an alternative approach to create volumetric and physiologically relevant tissue constructs. Therefore, the integration of scaffolds and microfluidics offers a promising platform, for creating accurate and predictive models of the skeletal muscle niche. Opening new avenues for basic research, drug development, and also tissue engineering.
Why the 2PP 3D printing was the best choice for creating the hybrid microfluidic-culture system mould?
For the creation of such hybrid system, 2PP 3D printing was used because it offers several advantages over other microfabrication methods (see table). These advantages mainly derive from the ability of 2PP to produce high-resolution structures with complex geometries and multiscale features, which are crucial for the functionality of the device.
Microfabrication methods | Pro | Cons |
Micro-milling (PMMA) |
|
|
Photolithography |
|
|
2PP 3D Printing |
|
|
More in details, the main advantages of 2PP 3D printing are:
1. Resolution and Accuracy
2PP 3D printing offers µm resolution, allowing for the creation of sensitive microfluidic features, such as the capillary valve array (figure2), with high precision. Other methods, such as micromilling, have resolution limitations that can compromise the dimensional accuracy of such features. This dimensional accuracy is critical for the proper functioning of the capillary valve, which relies on precise geometric dimensions to create the fluidic compartmentalization necessary for co-culture.
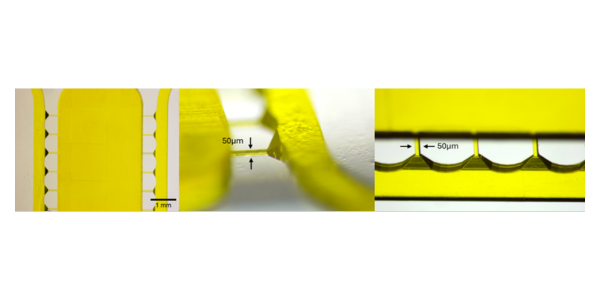
2. Ability to create slopes
2PP 3D printing can fabricate features with different heights and integrate sloped regions in a single process (figure1). This capability is beneficial for creating smooth transitions between different sections of the device, such as between inlet/outlet ports and channels. Sloped regions at the ports help reduce local flow forces and improve cell adhesion in these critical areas. Traditional methods, such as photolithography, require multiple passes to create structures with different heights, making the fabrication process more complex and error-prone. Moreover is impossible to create slope with such technique.
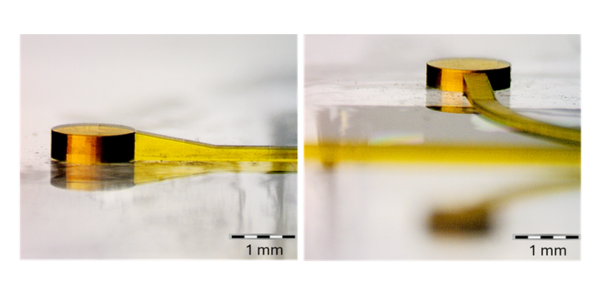
Figure 2: slope at the inlet and outlet of the microfluidic device obtained via 2PP 3D printing
3. Design Flexibility
2PP 3D printing enables the creation of complex geometries and 3D features, which are not easily achievable with other microfabrication methods. This design flexibility is particularly important for the hybrid system, which combines structures at the micro- and meso-scale with precise control over dimensions and geometries is particularly beneficial for tissue engineering.
4. Enhanced Scaffold Design
Additive manufacturing can produce organized and aligned hydrogel scaffolds, which are critical for mimicking the natural structure of skeletal muscle tissues. This method supports the fabrication of scaffolds that promote cell alignment and tissue organization, crucial for functional muscle constructs.
2PP 3D printing, despite its relative high cost, offers a unique combination of high resolution, design capability, and replicability that makes it the best choice for creating the hybrid microfluidic-culture system. Its ability to create complex geometries with dimensional accuracy is critical for the proper functioning of the device and its ability to support the culture and interaction of different cell types.
The impact of the design, obtained exclusively through 2PP 3D printing, on cell experiments
As highlighted, 3D printing based on 2PP technology facilitates the fabrication of devices that can present structures with intricate details and micrometer resolution. These features can improve cell alignment and tissue organization. Specifically in this study, 3D printing was used to fabricate the master mold for the microfluidic device, which includes critical elements for cell alignment such as:
Sloped regions at the inlets and outlets:
These regions were integrated to reduce local flow forces and improve cell adhesion. The ability of 2PP to create sloped geometries is critical to the realization of these features, which are impossible to achieve with traditional fabrication techniques.
Capillary channels:
2PP enabled the fabrication of channels with cross-sections of only 50 x 50 µm. These microchannels were used as a capillary valves. which are essential for creating a controlled and compartmentalized cell culture environment, which allows studying complex cellular interactions and developing in vitro models of more physiologically relevant tissues.
The final mould in figure 3 was printed in less than 15 hours, which is really impressive for the dimension, and the structural peculiarity shown by this design (maximum height: 400µm).
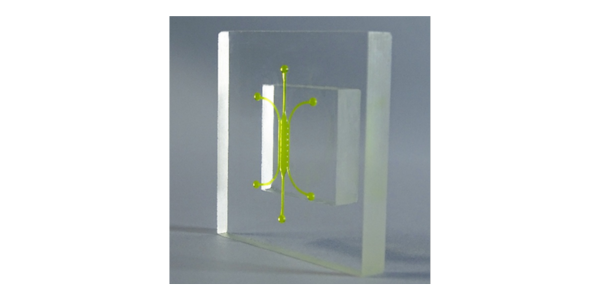
Figure 3: 2PP 3D printing master mould
The hybrid microfluidic device enabled the culture of large three-dimensional muscle tissue constructs, resulted in the formation of multinucleated myofibers, indicating successful cell differentiation (figure4).
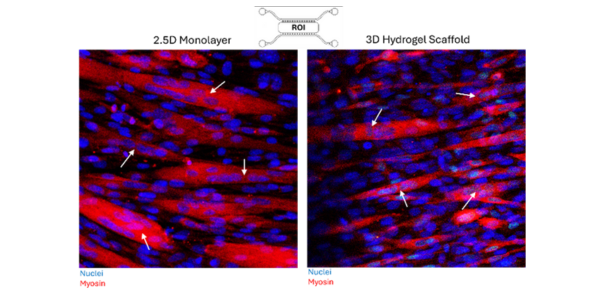
Figure 4: Immunofluorescent microscopy images of both 2.5D and 3D C2C12 multinucleated myofibres. The ROI (region of interest) is the central chamber where the muscle construct is cultured. Arrows indicate multinucleated regions, DAPI (blue) and myosin marker MF20 (red), magnification 30X. Samples were fixed on day 5 – 7 after seeding,
The 3D platform demonstrated significantly better alignment of myofibers compared to the 2.5D platform (figure5). This result was attributed to the fact that the 3D environment of the hydrogel provides more physiologically relevant cues for muscle tissue maturation and organization.
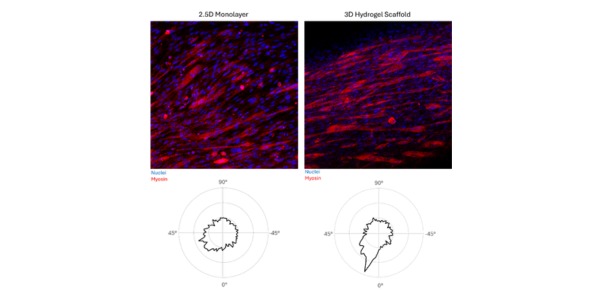
Figure 5: Directional alignment of 2.5D and 3D C2C12 cultures. Averaged across the z-stack from magnification 10X confocal images. DAPI stain for the nuclei (blue) and myosin marker MF20 (red). Samples were fixed on day 5 – 7 after seeding.
Conclusion
3D printing stands out as a transformative tool in skeletal muscle engineering, providing unmatched precision, customization, and integration of complex features. By leveraging techniques such as 2PP and bioprinting, researchers can develop advanced scaffolds, and microfluidic devices that overcome traditional fabrication limitations. The potential applications of 3D printing in creating functional in vitro models and therapeutic platforms are vast. Promising significant advancements in biomedical research and clinical therapies. For this specific study, the use of 3D printing via 2PP enabled the fabrication of a device, with high precision and intricate details, crucial for the success of cell culture experiments. The device allows the culture of aligned 3D muscle constructs, the application of controlled mechanical stimuli, and co-culture with other cell types, opening new perspectives for skeletal muscle research.
In conclusion, the choice of the most suitable microfabrication technique depends on the specific requirements of the device and also on the available resources, however 2PP 3D printing stands out for its ability to create high-resolution structures with complex geometries, with relative high costs and long production times.