From Organ-on-Chips to Body-on-a-Chip
With the help of microfluidics, cell culture is taking the next steps with Organ-on-Chips technology.
To highlight these incredible milestones, here we discuss a recent Nature review by Tagle et al.[i], members of the National Center for advancing Translational Sciences; We focus on the benefits of the field, discuss its challenges, and explain how Eden Tech can contribute to the advancement of Organ-on-Chip microfluidic devices for cell culture.
In recent decades, remarkable advances in our understanding of histology and organ organization have allowed us to model in vitro human organs. But for what purpose? The ultimate goal is to find a better alternative to animal models, in terms of ethics, accuracy, convenience, regulation and cost.
Regulatory pressures
Thanks to governmental pressure, the principles of the 3Rs (Replacement, Reduction and Refinement) were developed to limit animal use for research[ii], and the European REACH regulation were created in 2007 to ensure protection of human health and the environment against the effects of all chemical compounds in Europe (including Pharmaceuticals). These measures seek to improve our understanding of the dangers of chemicals, while avoiding unnecessary animal testing. Registrants, such as pharmaceutical companies, can only perform new tests when they have exhausted all other available relevant data sources[iii]. Furthermore, the European cosmetic regulation n ° 1223/2009 stipulates that cosmetic products cannot be marketed if their final formula, their development prototypes, or their ingredients have been tested on animals.
Animal model Relevance
Organ-on-Chips: a promising alternative to animal models
An organ on a chip (« OoCs ») is a miniaturized system that is designed using the architecture of a human organ to mimic its physiological functions.[iv] The use of microtechnology, and especially microfluidics, makes it possible to perfuse cellular structures and to control the physical and biochemical environment of the cells in order to obtain the desired multicellular architecture. The first « lung on a chip » platform developed was published in 2010 by Huh et al. The authors managed to recreate the alveolar-capillary interface of lungs, and mimick the breathing motion by inducing stretch forces. It allowed them to replicate complex in vivo responses (such as inflammation, and response to infection)[v].
Since then, a multitude of 3D in vitro models have been developed to reproduce organ functions, and can be categorized into two groups: regular Organ-on-Chips in microfluidic devices, and organoids simulating “mini organs” or “mini tumors”. The latter are obtained from stem or progenitor cells placed in 3D culture in hydrogel, which mimics the extracellular matrix without a microfluidic architecture. Co-cultures of different cell types are generally used. The concept of organoid is to let the cells organize themselves into functional tissue, mainly by playing with the stimulants in the culture medium[vi]. This model gives low control over the cell differentiation and organization which can induce high variability in the results.
Organ-on-Chips
- Grow on microfluidic chips
- Controlled designed
- Require microfabrication
- Cell maturity and ECM interactions
- Perfusion by microfluidic channels
(+/- endothelial vessels) - Low variability, high relevance
Organoids
- Grow on Hydrogel
- Self-organization (Low control on cell architecture)
- Few technical challenges
- ECM interactions
- Possible spontaneous vascularisation
- High variability of results
Key Features of Organ-on-Chips vs. Organoids
New devices are now available, capable of providing cells with an environment that is adapted to their growth and guides their differentiation, making it possible for innovators that are new to microfluidics to develop organs on chips. Equipped with micro-sensors for multi-parameters and highly parallelized monitoring. These Organ-on-Chips are made to mimic human physiology like mini-organs [vii].
However, many improvements are still required for such technologies to get closer to nature – namely on cell sourcing, medium, cell scaffolds, and general architectural designs, among others…
Precise 3D organization is required for proper differentiation of the cells because it affects their polarity, morphology, and interactions. To build Organ-on-Chips, we can use decellularized scaffold or seed cells on hydrogels, which are biocompatible polymers that allow cell adhesion[viii].
Each organ or part of an organ has its structural specificity that needs to be understood and modeled. For example, in 2018, Li and colleagues build an acinus of a liver in a glass and collagen architecture. They manage to create three vascularized zones with different levels of oxygenation, designed for modeling liver diseases and lead ADMET trials (Administration, distribution, metabolization and toxicology).[ix] Those trials are commonly used to study pharmacokinetics and toxicologic parameters of drug in preclinical trial, prior animal testing.
Next Step of Organ-on-Chips
We can already observe the potential of Organ-on-Chips as a powerful tool to study specific diseases or drug toxicity. But it is a technology in continuous evolution. For example, one of the most recent breakthroughs are « You-on-a-chip » where organs on chips are built with specific patient cells. The goal of this technology is to enable chemotherapy testing on tumors and provide patients with a personalized treatment[x].
Another challenge is to link several chips together to study “organ to organ” interactions. A team at the University of Chicago built an entire female reproductive system to mimic the menstrual cycle or pregnancy in order, to study reproductive toxicity. They linked 5 organs (ovary, cervix, uterus, fallopian tubes, and liver) in a system containing channels and micro-valves that irrigate the system[xi].
Consequently, we can observe that Linking multiple platforms requires a technological prowess and involves many challenges: the maintenance of sterility, the need for universal medium, the precise control of flow rate, and air bubble management. The fabrication of a full body on a chip is not going to happen overnight.
Technical challenges of Organ-on-Chips
Microfluidic devices are made of channels of sizes comparable to blood microvessels, which provide tissues with the nutrients and oxygen necessary for their proper function. Moreover, the flow circulating in these microchannels can induce biomechanical forces, to mimic the pressures exerted by vascularization. Those forces are necessary to induce the differentiation of cells. Thus, chip design must carefully model tissue forces by playing on channel diameters, corners and choice of materials which can influence flow[xii]. In addition, communication between “organs” must be designed to maintain the sterile condition necessary for cell culture, while allowing renewal of culture medium. They must also be adapted for sample collection necessary for the study.
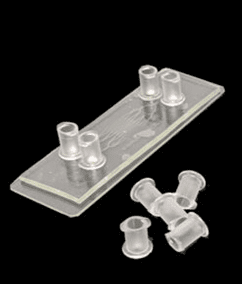
Eden Tech Connectors, to connect microfluidic devices to pumps. Luer Lock adaptors are combined with O-ring adhesives tested for cytotoxicity.
For instance, polydimethylsiloxane (PDMS), a biocompatible silicon-based polymer commonly used in microfluidics, allows prototyping of cell culture chips. PDMS is optically transparent and ideal for real time tissue imaging. However, it is gas permeable (which may or may not be an advantage) and exhibits high absorbance of small hydrophobic molecules[xiii]. Therefore, PDMS can be problematic for drug studies, because it can absorb a large amount of drugs, or other molecules released by cells. There is also a risk of cross contamination between adjacent channels. Therefore, steps should be included for treating PDMS surfaces to prevent cell adhesion or drug loss [xiv][xv][xvi].
FlexdymTM, which is also a biocompatible and transparent polymer, has the advantage of being easier to handle and less expensive than PDMS. In contrast, its prototyping can be done by hot embossing outside a clean room, with a simple and straight forward protocol. The fabrication of the microfluidic device and its replication can be achieved using the Sublym hot Embossing machine, taking only a few minutes. Finally, the assembly does not require specific treatment like harsh temperatures or chemicals [xvii]. FlexdymTM is highly flexible and stretchable material (elongation up to 700%), which allows the modulization of bioforces.
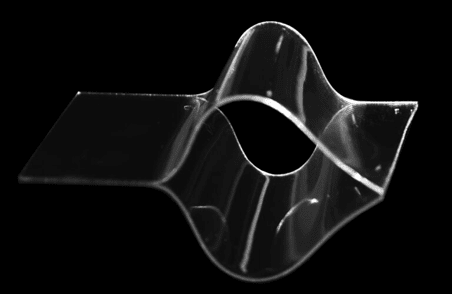
A FlexdymTM sheet of 1200µm thickness
With the vast applications of organ-on-chips, the choice of the material is critical in the early stage of research and development. Especially if the final goal is the industrialization of the prototypes. In these cases, FlexdymTM can be a real advantage, because it is a thermoplastic elastomer that is compatible with mass manufacturing processes. This eases the transition of the microfluidic technology from research to market.
1) Organ-on-Chips in Fundamental Research. Organ-on-Chips can make it possible to discover new information about the essential molecules and parameters that need to be controlled, to mimic physiological functions of organs. The study of these functions will thus complete our knowledge of pathophysiological processes that can affect organs[xviii], 2), 3) Drug screening and Drug development. Organ-on-Chips may also provide new methods to study drug candidates capable of correcting a dysfunction. Finally, they may also help to better understand the mechanism of action of a pharmacological molecule, necessary for the marketing of any new drug; and help with personalized therapeutic choices. For example, in oncology, cells from a patient’s tumor biopsy can be used to make organoids or tumor-on-chips. This can then allow us to test for the most effective molecules in treating the tumor. Such methods are already being applied in the field of 4) Personalized Medicine, and 5) Regenerative Medicine. Although these are more distant prospects, the controlled and reproducible manufacture of microfluidic devices as functional organ substitutes, will represent a significant advancement for various medical fields, such as regenerative medicine, where the purpose is to replace or repair a failing organ.[xix]
References
[ii] Directive 2010/63 / EU of the European parliament and of the council of 22 September 2010
[iii] Article 117(3) of the REACH Regulation
[iv] SN. Bhatia and DE. Ingber Microfluidic organs-on-chips. Nature Biotechnology. 2014; 32:760–772
[v] D. Huh, et al. Reconstituting organ-level lung functions on a chip. Science. 2010; 328:1662–1668
[vi]G. Rossi, et al. Progress and potential in organoid research. Nature Reviews Genetics. 2018
[vii] Low LA, Mummery C, Berridge BR, Austin CP, Tagle DA. Organs-on-chips: into the next decade. Nat Rev Drug Discov. 2020 Sep 10. doi: 10.1038/s41573-020-0079-3. Epub ahead of print. PMID: 32913334.
[viii] Crapo, P. M., Tottey, S., Slivka, P. F. & Badylak, S. F. Effects of biologic scaffolds on human stem cells and implications for CNS tissue engineering. Tissue Eng. Part. A 20, 313–323 (2013).
[ix] Li, X., George, S. M., Vernetti, L., Gough, A. H. & Taylor, D. L. A glass-based, continuously zonated and vascularized human liver acinus microphysiological system (vLAMPS) designed for experimental modeling of diseases and ADME/TOX. Lab Chip 18, 2614–2631 (2018).
[x] Shirure, V. S. et al. Tumor-on-a-chip platform to investigate progression and drug sensitivity in cell lines and patient-derived organoids. Lab Chip 18, 3687–3702 (2018)
[xi] Xiao, S. et al. A microfluidic culture model of the human reproductive tract and 28-day menstrual cycle. Nat. Commun. 8, 14584 (2017).
[xii] Stone, H. A., Stroock, A. D. & Ajdari, A. Engineering flows in small devices: microfluidics toward a lab-on-a-chip. Annu. Rev. Fluid Mech. 36, 381–411 (2004).
[xiii] Toepke, M. W. & Beebe, D. J. PDMS absorption of small molecules and consequences in microfluidic applications. Lab Chip 6, 1484–1486 (2006).
[xiv] Markov, D. A., Lillie, E. M., Garbett, S. P. & McCawley, L. J. Variation in diffusion of gases through PDMS due to plasma surface treatment and storage conditions. Biomed. Microdevices 16, 91–96 (2014).
[xv] van Meer, B. J. et al. Small molecule absorption by PDMS in the context of drug response bioassays. Biochem. Biophys. Res. Commun. 482, 323–328 (2017)
[xvi] Chuah, Y. J. et al. Simple surface engineering of polydimethylsiloxane with polydopamine for stabilized mesenchymal stem cell adhesion and multipotency. Sci. Rep. 5, 18162 (2015).
[xvii] Tan, S. H., Nguyen, N.-T., Chua, Y. C. & Kang, T. G. Oxygen plasma treatment for reducing hydrophobicity of a sealed polydimethylsiloxane microchannel. Biomicrofluidics 4, 032204 (2010).
[xviii] S. Bian, et al. Genetically engineered cerebral organoids model brain tumor formation. Nature Methods. 2018; 631-639.
[xix] Xavier Gidrol, B Laperrousaz, Patricia Obeid, S Porte, S Gerbeau, et al. Organoids-based High Content Screening in Oncology. BioBeirut 7 International Meeting, Nov 2017, Beyrouth, Lebanon. ⟨hal-02110699⟩
Dr. Constance Porrini
Eden R&D MedTech, Scientific Writer
PharmD. and Ph.D. in Microbiology from National Research Institute for Agriculture, Food and the Environment (INRAE)